top of page
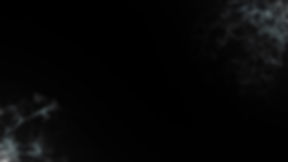
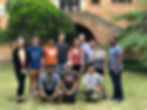

Matouschek Lab

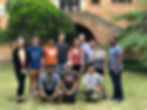
1/9
Understanding Biological Machines
The Matouschek lab is broadly interested in proteostasis. Our main focus is the ubiquitin proteasome system, the principal mechanism by which eukaryotes selectively degrade proteins. Much of the lab's research involves aspects from biochemistry, molecular biology, structural biology, and synthetic biology in order to understand how our cells regulate the protein landscape. Our overarching goal is to apply this information to technology development and human health.
Recent Lab News
November 17, 2020 - Caroline defends her dissertation, congrats Dr!
October 9, 2020 - Frank's last day!
September 1, 2020 - Takuya's last day!
Click here for more news from the lab!
bottom of page